The term "weakest material in the world" might conjure up images of delicate, brittle substances that crumble at the slightest touch. In the world of materials science, however, the concept of weakness is more complex than it appears. It encompasses factors such as tensile strength, compressive strength, and bonding properties, all of which contribute to a material's overall integrity and performance. Understanding these weak materials can provide valuable insights into the limitations and applications of different substances in various industries.
While materials like steel and titanium are celebrated for their strength and durability, the weakest materials often go unnoticed. Yet, these materials play crucial roles in specific applications where flexibility, lightness, or specific chemical properties are required. For instance, in the world of nanotechnology and electronics, certain weak materials are indispensable due to their unique characteristics. Furthermore, studying these materials helps scientists to develop new composites and alloys that can overcome inherent weaknesses, paving the way for innovative solutions and advancements in technology.
In this article, we will delve into the fascinating world of the weakest materials, exploring their properties, uses, and the scientific principles that define their limitations. From natural materials like aerogels to man-made synthetics, we will examine a variety of substances, providing a comprehensive overview that is both informative and engaging. Join us on this journey as we uncover the intricacies of these often-overlooked materials and their significance in the broader context of material science.
Table of Contents
- Understanding Weakness in Materials
- Aerogels: The Lightest Solids
- Graphene Oxide: A Compromise of Strength
- Biomaterials in Nature
- Synthetic Polymers and Their Limitations
- Mica and Its Fragility
- The Role of Weak Materials in Technology
- Comparison of Material Strengths
- Future Prospects in Material Development
- Innovations Deriving from Weak Materials
- Case Studies in Material Weakness
- Environmental Impact of Weak Materials
- FAQs
- Conclusion
Understanding Weakness in Materials
Before delving into specific materials, it's crucial to understand what defines weakness in materials science. Weakness is typically associated with a lack of strength under mechanical stress, which includes both tensile strength (the resistance to being pulled apart) and compressive strength (the resistance to being squeezed or compacted). Additionally, a material's bonding properties, density, and structural integrity all contribute to its perceived weakness.
Materials that are considered weak may have low tensile strength, making them susceptible to tearing or breaking when force is applied. This property is often seen in materials with loose molecular structures or weak intermolecular bonds. Compressive strength, on the other hand, refers to the ability of a material to withstand pressure without undergoing deformation. Materials with low compressive strength may crumble or compress easily under weight.
Another critical aspect of weakness is the material's elasticity and flexibility. Some materials, while weak in terms of strength, may exhibit significant elasticity, allowing them to bend or stretch without breaking. This characteristic is particularly valuable in applications that require materials to absorb impacts or accommodate movement without sustaining damage.
Overall, the concept of weakness in materials is multifaceted and context-dependent. While it may seem like a disadvantage, weak materials can offer unique benefits in specific applications, where their particular properties are advantageous. Understanding this complexity is essential for material scientists and engineers when designing and selecting materials for various purposes.
Aerogels: The Lightest Solids
Aerogels are a group of materials known for their extraordinary lightness and low density. Often referred to as "frozen smoke" due to their translucent appearance and ethereal lightness, aerogels are among the lightest solid materials available. These materials are composed of a nanoporous structure that gives them their unique properties, including low thermal conductivity and high surface area.
The production of aerogels involves a process known as sol-gel, where a liquid precursor is transitioned into a gel and then dried without collapsing the gel structure. This drying process, known as supercritical drying, removes the liquid component while maintaining the structural integrity of the gel, resulting in a highly porous and lightweight solid.
Aerogels are primarily composed of silica, but they can also be made from other materials like carbon, metal oxides, and organic polymers. Despite their impressive properties, aerogels are inherently weak when it comes to mechanical strength. The same nanoporous structure that makes them light and insulating also makes them fragile and prone to cracking under stress.
Despite their mechanical weakness, aerogels are incredibly useful in applications requiring thermal insulation, such as in aerospace and building materials. Their ability to trap air within their structure makes them excellent insulators, and their lightweight nature is advantageous in minimizing load and weight in constructions.
In recent years, researchers have been exploring ways to enhance the mechanical strength of aerogels while preserving their other beneficial properties. By incorporating reinforcing agents or developing new composite formulations, scientists aim to create aerogels that can withstand greater mechanical stress, broadening their range of applications.
Graphene Oxide: A Compromise of Strength
Graphene, a single layer of carbon atoms arranged in a hexagonal lattice, is celebrated for its exceptional strength and conductivity. However, when this remarkable material undergoes oxidation, it transforms into graphene oxide, a material with altered properties and reduced strength. Graphene oxide is considered one of the weakest forms of graphene, yet it offers unique benefits that make it valuable in certain applications.
The oxidation process involves the introduction of oxygen-containing groups to the graphene lattice, which disrupts the pristine carbon structure and imparts new chemical functionalities. These changes result in a material that is more hydrophilic and easier to process in aqueous solutions. However, the introduction of defects and disruptions in the carbon lattice also compromises the strength and electrical conductivity of graphene oxide.
Despite its reduced mechanical properties, graphene oxide is highly sought after for its versatility and ease of modification. Its ability to disperse in water and form stable colloidal suspensions makes it a popular choice in the fabrication of composite materials, coatings, and thin films. Additionally, the functional groups on graphene oxide allow for chemical modifications, enabling the development of tailored materials with specific properties.
In the field of electronics, graphene oxide is utilized in flexible electronic devices, sensors, and energy storage systems. Its weak mechanical strength is offset by its flexibility and ability to form thin, transparent films, making it ideal for applications requiring lightweight and adaptable materials.
Researchers are continually exploring methods to overcome the limitations of graphene oxide by reducing its oxygen content or combining it with other materials to enhance its mechanical properties. These efforts aim to expand the potential applications of graphene oxide, leveraging its unique characteristics while addressing its inherent weaknesses.
Biomaterials in Nature
Nature is home to a vast array of biomaterials that exhibit varying degrees of strength and weakness. These materials, derived from living organisms, often display unique properties that evolved to fulfill specific biological functions. While some biomaterials are renowned for their strength, such as spider silk and bone, others are notably weak, yet they serve essential roles in the natural world.
Fungi, for example, produce materials like chitin, a polysaccharide that forms the exoskeletons of arthropods and the cell walls of fungi. Although chitin is relatively weak compared to other structural materials, its lightweight and flexible nature makes it suitable for protecting delicate organisms and providing structural support.
Another example of a weak biomaterial is cellulose, the primary component of plant cell walls. Cellulose is a polysaccharide composed of glucose units linked together in a linear chain. While individual cellulose fibers are weak, plants use a combination of cellulose, hemicellulose, and lignin to create a composite material that provides sufficient strength and rigidity for structural support.
In the aquatic realm, materials like nacre, or mother-of-pearl, are synthesized by mollusks. Nacre is composed of aragonite, a form of calcium carbonate, arranged in a highly ordered structure that imparts toughness and resilience. Despite its brittleness, nacre's unique microstructure allows it to absorb and dissipate energy, making it an effective protective layer for mollusks.
The study of natural biomaterials provides valuable insights into designing and engineering synthetic materials with similar properties. By understanding the mechanisms and structures that contribute to the strength and weakness of biomaterials, scientists can develop new materials for a wide range of applications, from biodegradable plastics to medical implants.
Synthetic Polymers and Their Limitations
Synthetic polymers, such as plastics and resins, are ubiquitous in modern life, offering versatility and affordability for a wide range of applications. However, not all synthetic polymers are created equal, and many exhibit inherent weaknesses in terms of mechanical strength and durability.
One of the most well-known weak polymers is polystyrene, a lightweight and inexpensive plastic commonly used in packaging and disposable products. While polystyrene offers excellent insulation properties and ease of processing, its brittleness and low impact resistance make it unsuitable for applications requiring high durability.
Another example is polyvinyl chloride (PVC), a widely used polymer known for its chemical resistance and versatility. Despite its widespread use, PVC is prone to degradation when exposed to UV light and high temperatures, leading to embrittlement and loss of mechanical properties over time.
In the realm of biodegradable polymers, materials like polylactic acid (PLA) and polyhydroxyalkanoates (PHA) are gaining popularity due to their eco-friendly nature. However, these materials often suffer from limited mechanical strength and thermal stability, restricting their use in demanding applications.
To address these limitations, researchers are exploring various strategies to enhance the properties of synthetic polymers. These include the development of polymer composites, the incorporation of reinforcing fillers, and the modification of polymer chains through chemical reactions. By tailoring the structure and composition of synthetic polymers, scientists aim to create materials that combine the benefits of flexibility, lightweight, and environmental sustainability with improved strength and durability.
Mica and Its Fragility
Mica is a naturally occurring mineral known for its distinctive sheet-like structure and excellent thermal and electrical insulating properties. It is composed of silicate minerals that can be split into thin, flexible sheets, making it valuable in various industrial applications. However, mica's unique structure also contributes to its fragility, making it one of the weaker materials in terms of mechanical strength.
The sheet-like structure of mica arises from its layered arrangement of silicate tetrahedra, which are held together by weak van der Waals forces. This structure allows mica to be easily cleaved into thin sheets, but it also makes the material prone to breaking and chipping under mechanical stress.
Despite its fragility, mica's excellent insulating properties make it indispensable in the electrical and electronics industries. It is used as an insulator in capacitors, transformers, and other electrical components, where its ability to withstand high temperatures and electrical fields is crucial.
In addition to its use in electronics, mica is also employed in the cosmetics industry, where its reflective and shimmering properties are harnessed in products like makeup and nail polish. Its natural occurrence in a variety of colors and its ability to be ground into fine powders make it a popular choice for adding luster and shine to cosmetic formulations.
To overcome mica's fragility, researchers are exploring methods to reinforce mica-based materials by combining them with other substances or developing composite materials. These efforts aim to enhance mica's mechanical properties while retaining its beneficial insulating and aesthetic qualities.
The Role of Weak Materials in Technology
In the ever-evolving world of technology, weak materials play a crucial role in enabling innovation and advancements across various fields. While strength is often a desirable attribute in materials, certain applications benefit from the unique properties offered by weaker substances. These applications leverage the flexibility, lightness, and specific chemical properties of weak materials to achieve functionality and efficiency.
One such application is in the realm of flexible electronics, where materials like graphene oxide, polypyrrole, and other conductive polymers are used to create bendable and stretchable devices. These materials, despite their limited mechanical strength, offer the flexibility and conductivity required for the development of wearable electronics, foldable displays, and flexible sensors.
In the field of aerospace, lightweight materials like aerogels and foams are employed to reduce the overall weight of aircraft and spacecraft, improving fuel efficiency and performance. These materials, though weak in terms of mechanical strength, provide excellent thermal and acoustic insulation, making them invaluable in demanding environments.
The field of biomedical engineering also benefits from weak materials, particularly in the development of tissue scaffolds and biodegradable implants. Materials like polylactic acid and chitosan are used to create structures that support cell growth and tissue regeneration, while their inherent weakness allows them to degrade naturally within the body over time.
In the realm of environmental technology, weak materials like biodegradable polymers and natural fibers are utilized to create sustainable alternatives to traditional plastics. These materials, while not as durable as conventional plastics, offer a reduced environmental impact and the potential for biodegradation, making them ideal for single-use products and packaging.
Overall, the role of weak materials in technology is multifaceted and essential. By harnessing their unique properties and addressing their limitations, scientists and engineers continue to push the boundaries of innovation, developing new technologies that benefit society and the environment.
Comparison of Material Strengths
Understanding the relative strengths and weaknesses of various materials is essential for selecting the appropriate material for a given application. The strength of a material is typically evaluated based on its tensile strength, compressive strength, and other mechanical properties, which are determined through standardized testing methods.
Tensile strength refers to a material's ability to withstand being pulled apart without breaking. Materials like steel and carbon fibers are known for their high tensile strength, making them ideal for applications requiring load-bearing capabilities, such as construction and automotive industries.
Compressive strength, on the other hand, measures a material's ability to withstand being compressed or squeezed. Concrete and ceramics are examples of materials with high compressive strength, making them suitable for structural applications where resistance to compression is critical.
In contrast, materials like aerogels and certain polymers exhibit low mechanical strength, both in tension and compression. These materials are often chosen for applications where weight reduction, flexibility, or specific chemical properties are more important than strength.
Another important aspect to consider is the material's elasticity and ductility. Elasticity refers to a material's ability to return to its original shape after deformation, while ductility refers to its ability to undergo significant deformation before breaking. Materials like rubber and certain metals are highly elastic and ductile, making them suitable for applications requiring flexibility and impact resistance.
By comparing the strengths and weaknesses of different materials, engineers and scientists can make informed decisions about material selection, ensuring that the chosen material meets the specific requirements of the application while balancing factors such as cost, performance, and sustainability.
Future Prospects in Material Development
The field of material science is constantly evolving, with researchers exploring new materials and technologies to address the challenges of the modern world. The development of materials with tailored properties, including those considered weak, holds great promise for future innovations and applications.
One area of focus is the development of advanced composites, which combine different materials to create a product with enhanced properties. By incorporating reinforcing agents, such as carbon nanotubes or nanocellulose, scientists aim to improve the mechanical strength and performance of traditionally weak materials, expanding their range of applications.
Another promising avenue is the exploration of bioinspired materials, which mimic the structures and functions of natural materials. By studying the unique properties of biomaterials like spider silk and nacre, researchers are developing synthetic analogs that offer similar benefits while overcoming the limitations of their natural counterparts.
In the realm of sustainable materials, efforts are being made to develop biodegradable and recyclable alternatives to conventional plastics. These materials, while often weaker than their synthetic counterparts, offer significant environmental benefits, reducing waste and pollution.
The advancement of nanotechnology also presents exciting opportunities for material development. By manipulating materials at the nanoscale, scientists can create materials with unprecedented properties and functionalities, paving the way for innovations in electronics, medicine, and energy.
Overall, the future of material development is bright, with ongoing research and innovation driving the discovery of new materials and technologies. By understanding and harnessing the unique properties of weak materials, scientists can continue to push the boundaries of what is possible, creating solutions that address the needs and challenges of the modern world.
Innovations Deriving from Weak Materials
Despite their inherent limitations, weak materials have been the driving force behind numerous innovations across various industries. By leveraging their unique properties, scientists and engineers have developed groundbreaking technologies and solutions that address specific challenges and requirements.
One notable example is the development of soft robotics, which utilizes flexible and deformable materials to create robots capable of navigating complex environments and interacting safely with humans. These robots, often made from materials like silicone and conductive polymers, offer advantages in terms of adaptability and safety, making them ideal for applications in healthcare, exploration, and manufacturing.
In the field of energy, weak materials like thin-film solar cells and organic photovoltaics are revolutionizing the way we harness solar energy. These materials, while not as efficient as traditional silicon-based solar cells, offer benefits in terms of cost, flexibility, and lightweight, enabling the development of innovative solar solutions for a wide range of applications.
The medical industry also benefits from innovations derived from weak materials, particularly in the development of drug delivery systems and tissue engineering. Materials like hydrogels and biodegradable polymers are used to create scaffolds and carriers that support cell growth and deliver therapeutic agents in a controlled manner, improving patient outcomes and treatment efficacy.
In the realm of sustainable packaging, weak materials like bioplastics and natural fibers are being utilized to create eco-friendly alternatives to conventional plastics. These materials, while not as durable, offer significant environmental benefits, reducing waste and promoting a circular economy.
Overall, the innovations derived from weak materials demonstrate the potential and versatility of these substances. By understanding their unique properties and addressing their limitations, scientists and engineers continue to push the boundaries of innovation, developing new technologies that benefit society and the environment.
Case Studies in Material Weakness
To better understand the implications and applications of weak materials, it is valuable to examine specific case studies that highlight their use and impact in various industries. These case studies provide insights into how weak materials are utilized and the challenges and opportunities they present.
One such case study is the use of aerogels in the aerospace industry. NASA has employed silica aerogels as insulation materials in spacecraft and rovers due to their lightweight and excellent thermal insulating properties. Despite their fragility, aerogels provide effective insulation in extreme temperature environments, contributing to the success of missions in space exploration.
Another case study involves the use of graphene oxide in the development of flexible and wearable electronics. Researchers have created sensors and devices that utilize graphene oxide's flexibility and conductivity to monitor various physiological parameters, such as heart rate and body temperature. These innovations demonstrate the potential of weak materials in advancing healthcare technology.
In the field of sustainable packaging, the use of polylactic acid (PLA) as a biodegradable alternative to conventional plastics is gaining traction. Case studies have shown that PLA-based packaging reduces environmental impact and supports a circular economy, highlighting the potential of weak materials in promoting sustainability.
In the medical industry, hydrogels have been used as scaffolds for tissue engineering and regenerative medicine. These materials, despite their limited mechanical strength, provide a supportive environment for cell growth and tissue regeneration, demonstrating their value in improving patient outcomes.
These case studies illustrate the diverse applications and benefits of weak materials across various industries. By understanding their unique properties and addressing their limitations, scientists and engineers continue to develop innovative solutions that address specific challenges and improve the quality of life.
Environmental Impact of Weak Materials
The environmental impact of materials is a critical consideration in material selection and development. Weak materials, particularly those that are biodegradable or derived from renewable sources, offer significant environmental benefits compared to traditional materials.
Biodegradable polymers, such as polylactic acid (PLA) and polyhydroxyalkanoates (PHA), are gaining popularity as sustainable alternatives to conventional plastics. These materials, while not as durable, degrade naturally in the environment, reducing waste and pollution. Their use in packaging, agriculture, and consumer products supports a circular economy and promotes sustainability.
Natural fibers, such as hemp, jute, and sisal, are also being used as eco-friendly alternatives to synthetic fibers in textiles and composites. These materials, which are derived from renewable sources, offer advantages in terms of biodegradability and reduced environmental impact, making them ideal for sustainable applications.
In the realm of construction, materials like straw bales and adobe, which are considered weak in terms of mechanical strength, are being utilized for their low environmental impact and energy efficiency. These natural building materials offer sustainability benefits, reducing the carbon footprint of construction projects.
However, the environmental impact of weak materials is not always positive. The production and disposal of certain weak materials, such as synthetic polymers, can contribute to pollution and environmental degradation. Efforts are being made to develop more sustainable production methods and recycling processes to mitigate these impacts.
Overall, the environmental impact of weak materials is a complex and multifaceted issue. By understanding their unique properties and addressing their limitations, scientists and engineers can develop materials that balance performance and sustainability, contributing to a more sustainable future.
FAQs
- What is the weakest material in the world?
The weakest material in the world is difficult to define as it depends on the context and specific properties being measured, such as tensile or compressive strength. However, materials like aerogels and certain polymers are often considered among the weakest due to their low mechanical strength.
- Why are weak materials important?
Weak materials are important because they offer unique properties such as flexibility, lightness, and specific chemical characteristics that are valuable in certain applications. They play crucial roles in industries like electronics, aerospace, and biotechnology.
- How are weak materials used in technology?
Weak materials are used in technology for applications that require flexibility, lightweight, and specific chemical properties. Examples include flexible electronics, thermal insulation in aerospace, and biodegradable polymers in packaging.
- Can weak materials be strengthened?
Yes, weak materials can be strengthened through various methods such as reinforcing with other materials, developing composites, or chemically modifying the material structure. These approaches enhance mechanical properties while retaining beneficial characteristics.
- What are some examples of weak materials in nature?
Examples of weak materials in nature include chitin in fungi and arthropods, cellulose in plant cell walls, and nacre in mollusks. These materials, while not particularly strong, serve essential biological functions and offer unique properties.
- How do weak materials impact the environment?
Weak materials can have both positive and negative environmental impacts. Biodegradable and renewable materials offer sustainability benefits, while some synthetic weak materials may contribute to pollution. Efforts are being made to develop more sustainable materials and processes.
Conclusion
In conclusion, the "weakest material in the world" is a concept that encompasses a wide range of materials with varying degrees of mechanical strength and unique properties. While these materials may lack the strength of traditional strong materials, they offer valuable benefits in specific applications, from flexible electronics to sustainable packaging. By understanding the complexities of weak materials and addressing their limitations, scientists and engineers continue to push the boundaries of innovation, developing new technologies that benefit society and the environment. As material science advances, the role of weak materials will continue to evolve, offering exciting possibilities for future developments and applications.
For further exploration of material science and innovations, consider visiting reputable sources like the Nature Materials Science Journal for the latest research and discoveries in the field.
You Might Also Like
The Unveiling Of Rafael Nadal's Wife: A Journey Through Life And LoveThe Significance And Interpretation Of Two Lines Tattoos On The Neck
A Comprehensive Guide To Simple Angel Wings Tattoo: Design, Meaning, And Cultural Significance
Andy Reid Weight Loss: The Inspiring Journey Of A Legendary Coach
Heartfelt Condolences For Sister: A Guide To Expressing Sympathy
Article Recommendations
- Gale Boeticher
- Anant Real Name
- Ihad Kroeger Married
- Michael C Hall
- Skarsg Rd Family
- Keri Russell And Kurt Russell Related
- Mingus Lucien Reedus
- Cassie Ventura Parents Nationality
- Michael Kitchen
- Jonathan Bailey Height
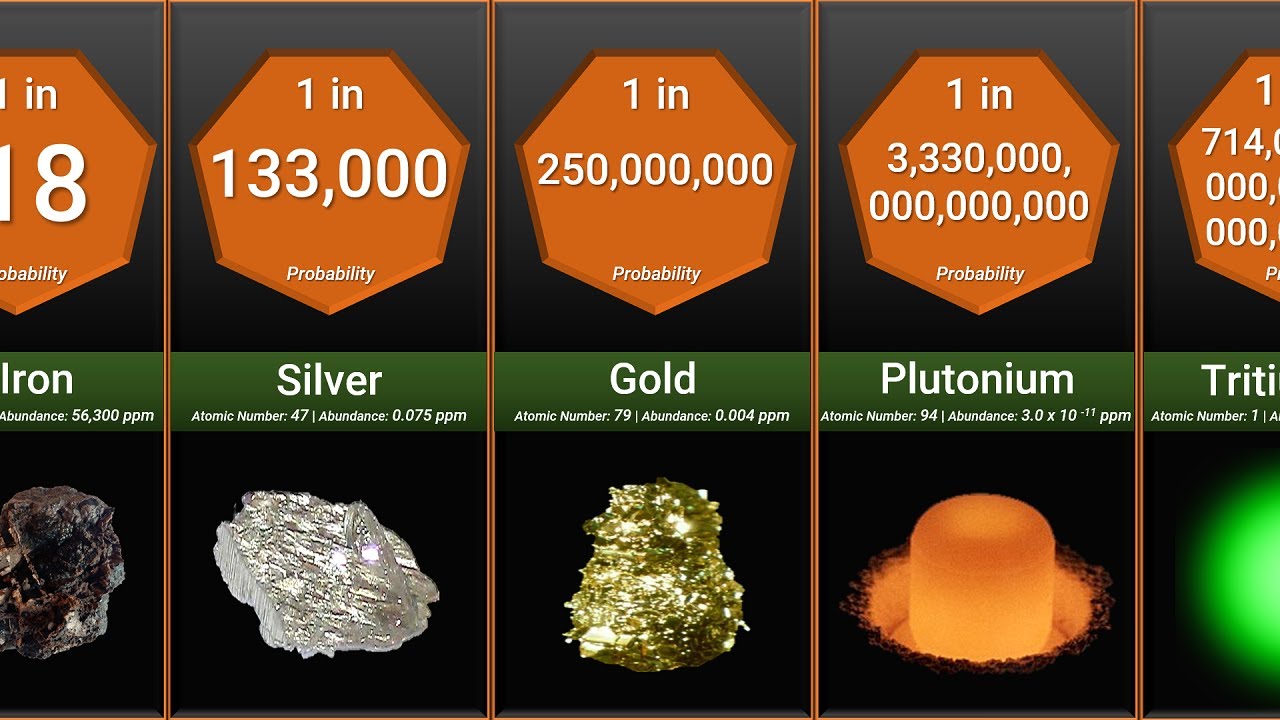
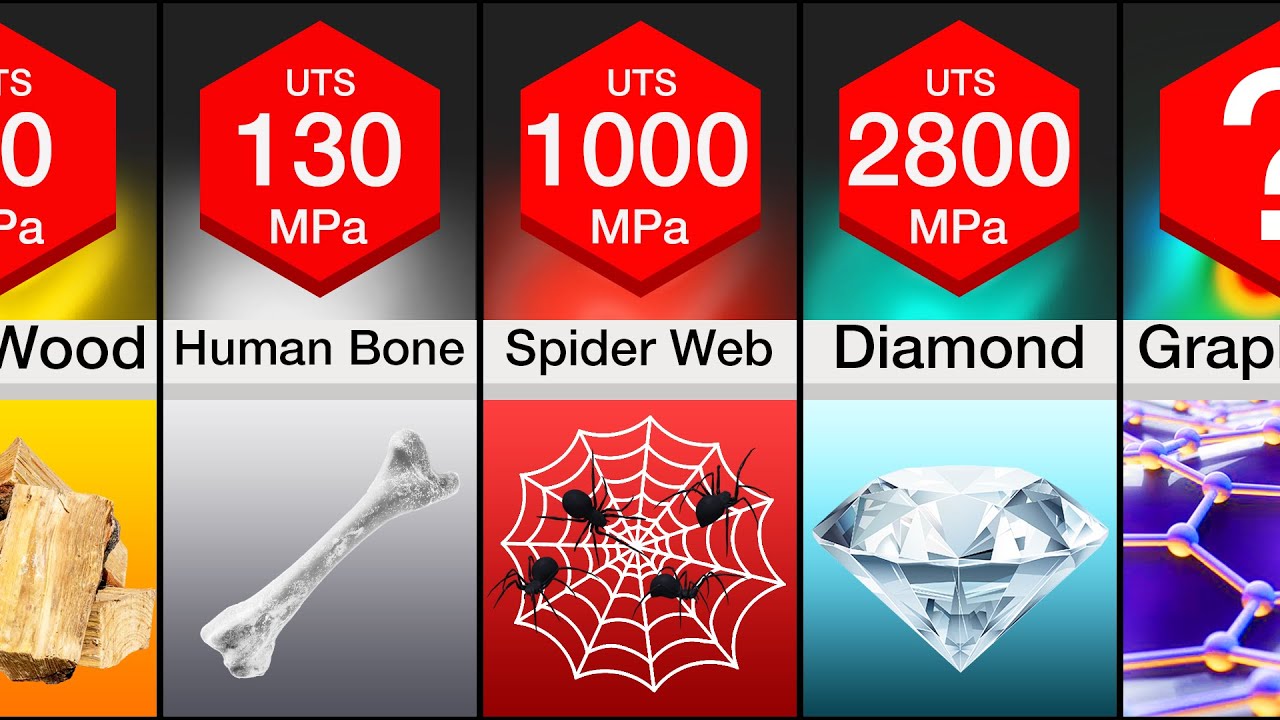